Vacuum Furnaces by MRC
Applications
Metal Additive Manufacturing (AM) is a process of building metal parts layer by layer using a 3D printer. This technology has the potential to replace traditional machined and grinded parts, as well as bring new possibilities in metal engineering for a variety of industries.
One of the main advantages of metal AM is its ability to create complex geometries and shapes that would be difficult or impossible to achieve using conventional manufacturing methods. This opens up new opportunities in industries such as aerospace, medical, and automotive where unique and highly specialized parts are required.
Additionally, metal AM offers faster production times, reduced waste, and lower costs compared to traditional manufacturing methods. This makes it ideal for small-batch production and prototyping, where quick turnaround and cost-effectiveness are essential.
Finally, metal AM allows for customization and personalization of parts, which is particularly relevant in the medical and dental industries where bespoke implants and prosthetics can be manufactured to meet the exact specifications of each patient.
Metal AM has the potential to revolutionize the way metal parts are manufactured and bring new possibilities in metal engineering across various industries.
Debinding and sintering are crucial steps in the process of metal additive manufacturing (AM).
Debinding is the process of removing the temporary binder that holds the metal powder together in the AM process. This binder is needed during the printing process to ensure the stability and accuracy of the printed part. However, once the printing is complete, the binder must be removed to prepare the part for the final manufacturing steps.
Sintering is the process of heating the metal powder to its melting point, causing the particles to fuse together and form a solid metal part. During sintering, the metal powder undergoes a phase transition from a loose powder to a solid, dense metal part. Sintering is a crucial step in the AM process because it determines the final mechanical properties, such as strength and density, of the finished part.
Debinding and sintering are usually performed in specialized furnaces designed specifically for these processes(HTK Serie). These furnaces must be able to maintain very precise temperature and atmosphere controls to ensure that the parts are manufactured to the highest quality standards. The furnaces must also be able to handle the high temperatures and corrosive environments that are required for these proceses.The inner chamber can be made from metal,graphite or ceramic and permit high purity of inert atmosphere( protective gases like Nitrogen/Argon, and reactive gases like Hydrogen and Carbon Monoxide) and high vacuum in the region of (5 x 10-6 mbar),and maximum temperature 2200 °C and 1600 °C.
Debinding and sintering furnaces are essential components of the metal AM process, and their use is required to ensure the quality and reliability of the final manufactured parts.
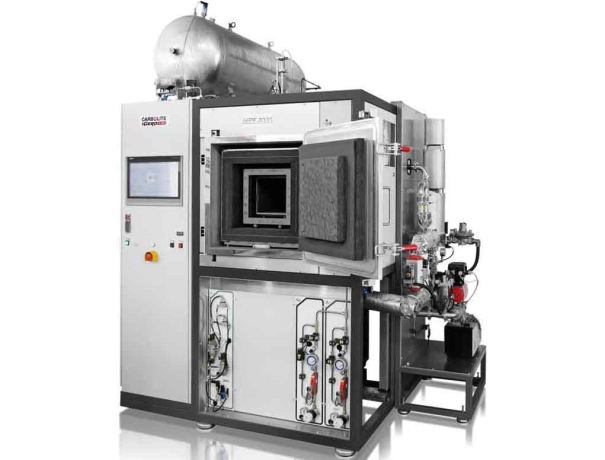
Metal Injection Moulding (MIM) that replaces machined and grinded parts and the new possibilities in metal engineering for all kind of industries.
Metal Injection Moulding (MIM) is a manufacturing process that involves the injection of metal powders into a mold, followed by sintering to produce a finished metal part. This process has the potential to replace traditional machined and grinded parts and bring new possibilities in metal engineering for various industries.
One of the main advantages of MIM is its ability to produce highly complex and intricate metal parts with tight tolerances, making it an ideal solution for industries such as medical devices, firearms, and electronics where precision is essential.
MIM also offers a cost-effective solution for small-batch production, as it eliminates the need for secondary machining and finishing operations. The process also offers significant material savings, as the metal powders used in MIM can be recycled, reducing waste and lowering costs.
Another advantage of MIM is its ability to produce parts with consistent mechanical properties and high density, which is crucial for applications where performance and durability are important, such as in the aerospace and automotive industries.
Finally, MIM allows for the production of parts with a wide range of metal alloys, including stainless steel, titanium, and nickel-based superalloys, making it a versatile manufacturing option for many industries.
Furnaces for the process of Metal Injection Moulding (MIM):
Debinding, sintering, and siliconization are important steps in the process of Metal Injection Moulding (MIM), and specialized furnaces are needed to carry out these processes.
Debinding is the process of removing the binder material used to hold the metal powders together during injection moulding. The binder is typically a polymeric material that must be removed before sintering. Debinding furnaces are designed to safely and effectively remove the binder while preserving the shape of the molded part.
Sintering is the process of heating the molded part to its melting point, causing the metal powders to fuse together and form a solid metal part. Sintering furnaces must be able to maintain precise temperature and atmosphere controls to ensure that the parts are sintered properly and achieve the desired mechanical properties, such as strength and density.
Siliconization is an optional step in the MIM process that involves the deposition of a thin silicon layer on the surface of the sintered part. This step can improve the surface properties of the part, such as reducing friction and improving wear resistance. Siliconization furnaces are designed to deposit a uniform and consistent layer of silicon on the sintered part.
Debinding, sintering, and siliconization furnaces are essential components of the MIM process, and their use is necessary to ensure the quality and reliability of the final manufactured parts. These furnaces must be designed to meet the specific requirements of the MIM process, including temperature and atmosphere control, to produce parts that meet the desired specifications and performance requirements.
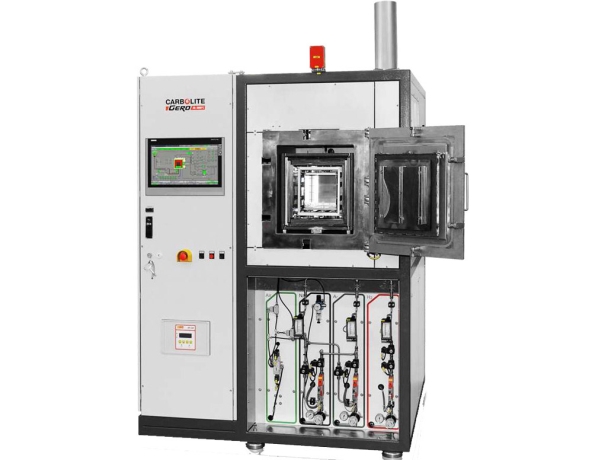
Technical ceramics, also known as advanced ceramics, are a group of materials with unique properties that make them suitable for use in various high-tech applications, including electronics, dental, medical, and others.
In electronics, technical ceramics are used as insulators and high-temperature substrates, due to their exceptional thermal stability and high electrical resistance. They are also used in the manufacture of components such as capacitors, inductors, and resonators, which are essential in a range of electronic devices, from smartphones to satellites.
In dental and medical applications, technical ceramics are widely used for their biocompatibility and toughness. Dental ceramics, for example, are used to make crowns, bridges, and implants, as they have a similar appearance to natural teeth and are resistant to wear and staining. Medical ceramics are also used in orthopedic and spinal implants, as they are lightweight and durable, making them ideal for long-term use.
Technical ceramics are also used in high-performance applications, such as aerospace, where their strength and thermal resistance make them ideal for use in components exposed to extreme temperatures and harsh environments. They are also used in energy applications, such as fuel cells and solar panels, where their high-temperature stability and electrical conductivity make them an ideal choice for various components.
Technical ceramics play a critical role in a range of high-tech applications, due to their unique properties and performance characteristics. Whether in electronics, dental, medical, or other applications, technical ceramics are a versatile and highly effective solution for many engineering challenges.
Furnaces for the process of making technical ceramics
Debinding, sintering, and siliconization furnaces are essential components of the process of making technical ceramics.
Debinding is the process of removing the binder material used to hold the ceramic powders together during the forming process. The binder must be removed to allow for proper sintering of the ceramic powders, as it can have a negative impact on the final properties of the ceramic. Debinding furnaces are designed to safely and effectively remove the binder, while preserving the shape and integrity of the ceramic part.
Sintering is the process of heating the ceramic part to its melting point, causing the ceramic powders to fuse together and form a solid ceramic part. Sintering furnaces must be able to maintain precise temperature and atmosphere controls, as the success of the sintering process is critical to the final properties of the ceramic part, such as its strength, density, and dimensional stability.
Siliconization is an optional step in the technical ceramics process that involves the deposition of a thin silicon layer on the surface of the sintered part. This step can improve the surface properties of the ceramic part, such as reducing friction and improving wear resistance. Siliconization furnaces are designed to deposit a uniform and consistent layer of silicon on the sintered ceramic part.
The high purity high vacuum heat treatment, soldering, and brazing market is a growing segment of the manufacturing and materials processing industries. This market is driven by the need for high-performance, high-quality, and reliable components in a range of applications, including electronics, aerospace, and medical devices.
High purity high vacuum heat treatment is a specialized process used to produce high-quality metal and ceramic components, using high temperatures and precise atmospheric conditions. This process is critical for the production of high-performance electronic components, such as semiconductors, where the cleanliness and purity of the material are essential.
Soldering and brazing are related processes used to join two or more metal components together, by melting and fusing a filler metal at the joint. These processes are widely used in the electronics and aerospace industries, where they are used to join delicate and complex components, as well as to produce high-quality, reliable, and leak-tight joints.
The high purity high vacuum heat treatment, soldering, and brazing market is characterized by strong demand for high-performance components, and a growing need for advanced and reliable manufacturing processes. This market is expected to continue to grow, driven by the increasing demand for high-tech components, and the need for advanced manufacturing processes that meet the stringent requirements of modern applications.
The high purity high vacuum heat treatment, soldering, and brazing market represents a growing and important segment of the manufacturing and materials processing industries, and is expected to continue to grow, driven by the need for high-performance, high-quality, and reliable components in a range of applications. These processes are critical to the production of advanced components and devices, and play a critical role in ensuring the quality and reliability of modern technologies.
Furnaces are needed in the soldering and brazing process to provide a controlled environment for the heating and joining of metal components. Furnaces provide the necessary heat to melt and fuse the filler metal, as well as control the temperature and atmosphere to ensure a high-quality and reliable joint.
In soldering, the filler metal must be melted at a temperature that is below the melting point of the base metals, to avoid damaging or altering the properties of the base metals. Furnaces provide precise temperature control to ensure that the filler metal is melted at the correct temperature, and that the joint is formed under optimal conditions.
In brazing, the filler metal is melted at a higher temperature than in soldering, and the base metals are subjected to higher temperatures. Furnaces play a critical role in ensuring that the brazing process is controlled and consistent, providing the necessary heat and atmosphere to produce high-quality and reliable joints.
Furnaces also play an important role in the cleanliness of the soldering and brazing process, as the high vacuum environment in the furnace can minimize contamination, ensuring the purity and quality of the metal components and the filler metal.
In conclusion, furnaces are a critical component of the soldering and brazing process, providing the necessary heat, temperature control, and atmosphere to ensure high-quality and reliable joints. The use of furnaces in the soldering and brazing process is essential to meet the stringent requirements of modern applications, and to ensure the quality and reliability of the final product.
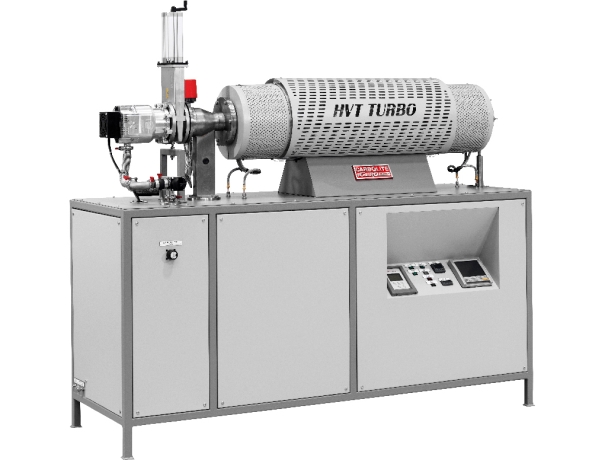
Crystal growth refers to the process of forming large, single crystals from smaller crystals or a melted material. Crystals are characterized by their highly ordered atomic structure, and they have unique properties that make them highly desirable for a wide range of high-tech applications.
Crystals have become increasingly important for many high-tech applications due to their unique properties, including high thermal stability, electrical conductivity, and optical transparency. For example, single crystal silicon is used as the substrate material for many semiconductor devices, such as computer chips and solar cells, due to its high electrical conductivity and thermal stability.
Single crystal growth is also critical for the production of advanced optical components, such as laser crystals, where the optical transparency and high thermal stability of the crystal are essential.
Crystals can be grown using various methods, including the Czochralski method, Bridgman method, and the Float Zone method. Each of these methods has its own advantages and disadvantages, and the choice of method will depend on the specific requirements of the application.
Crystals are highly valued for their unique properties, and their importance in many high-tech applications is expected to continue to grow. The development of advanced crystal growth techniques has enabled the production of high-quality, large single crystals, which are critical for the production of advanced semiconductors, optical components, and other high-tech applications. The growth of crystals is an important area of research and development, and is expected to play a critical role in the development of new and advanced technologies in the future.
תמונה HTRV-16-40-500
The Bridgman method is a crystal growth technique that utilizes a furnace to create high-quality, single crystals. Tube furnaces are an essential component of the Bridgman method, as they provide the necessary heat and temperature control to regulate the growth of the crystal. The melted material moves slowly through a decreasing temperature gradient and form a crystal.
The tube furnace mounted on the pulling device that moves the sample with an adjustable speed toward the lower temperature(bottom of furnace).
In the Bridgman method, the starting material, usually a boule or a powder, is placed in a crucible inside a furnace. The temperature of the furnace is then carefully controlled and increased to a temperature that is above the melting point of the material. The boule or powder is then slowly melted, and a seed crystal is introduced.
The seed crystal acts as a template, guiding the growth of the single crystal as the melted material slowly solidifies. The furnace is slowly cooled, and the temperature is carefully controlled to ensure that the crystal grows at a uniform rate.
The Bridgman method provides several advantages over other crystal growth methods, including the ability to grow large, high-quality single crystals and the ability to control the orientation of the crystal. The high temperature and controlled environment provided by the furnace are essential for the growth of high-quality single crystals using the Bridgman method.
Tube furnaces are an essential component of the Bridgman method of crystal growth, providing the necessary heat and temperature control to regulate the growth of the crystal. The use of furnaces in the Bridgman method enables the production of high-quality, large single crystals, which are critical for a wide range of high-tech applications.
Graphitization market:Carbon, Graphite and its derivatives are used in almost all Industries.
Carbonization and graphitization are important processes in the production of carbon and graphite materials, and their derivatives. Carbon materials, including carbon and graphite, have unique properties, including high thermal conductivity, high electrical conductivity, high mechanical strength, and chemical stability, which make them highly desirable for a wide range of industrial applications.
Carbonization refers to the process of heating organic materials, such as coal, wood, and petroleum coke, in the absence of air, to produce carbon materials. The resulting materials can be used in a wide range of applications, including as raw materials for the production of graphite and as fuel for power generation.
Graphitization, on the other hand, is the process of converting carbon materials into graphite. This is done by heating the carbon material to high temperatures in a controlled atmosphere, which causes the carbon atoms to rearrange themselves into a highly ordered, hexagonal lattice structure. The resulting graphite material has high thermal and electrical conductivity, high mechanical strength, and chemical stability, making it highly desirable for many industrial applications.
Graphite and its derivatives are used in almost all industries, including the electronics, energy, automotive, aerospace, and chemical industries. For example, graphite is used as an electrode material in the production of lithium-ion batteries, as a heat exchanger in nuclear reactors, and as a lubricant in high-temperature applications. Graphite derivatives, such as graphene, are also highly valued for their unique properties, including high electrical and thermal conductivity, high mechanical strength, and chemical stability, and are being used in a wide range of advanced applications, including as transparent conductors, in energy storage systems, and in biomedicine.
The carbonization and graphitization of carbon materials is a critical aspect of the production of carbon and graphite materials and their derivatives. These materials have unique properties, which make them highly desirable for a wide range of industrial applications, and their use is expected to continue to grow in the future.
Debinding is an important step in the carbonization and graphitization process of carbon and graphite materials. It is a process that is used to remove binders, such as wax, resin, or polymer, from a carbon-based composite material. The removal of binders is necessary before carbonization and graphitization can take place, as the presence of binders can interfere with the formation of the desirable carbon or graphite structure.
A debinding furnace is used to heat the carbon-based composite material to a high temperature in a controlled atmosphere, typically a reducing atmosphere, to remove the binders. The temperature and atmosphere in the furnace must be carefully controlled to ensure that the binders are removed while avoiding unwanted side reactions, such as oxidation or degradation of the carbon material.
The use of a debinding furnace provides several advantages over other debinding methods, including the ability to remove binders efficiently and uniformly, and the ability to control the temperature and atmosphere of the process. The controlled environment provided by the furnace is essential for the successful debinding of carbon-based composite materials.
In conclusion, debinding is an important step in the carbonization and graphitization process, and a debinding furnace is essential for the efficient and effective removal of binders from carbon-based composite materials. The use of a debinding furnace enables the production of high-quality carbon and graphite materials, which are critical for a wide range of industrial applications.
Pyrolysis is a thermal decomposition process that is used as a part of carbonization or graphitization. It involves heating organic materials, such as coal, wood, and petroleum coke, in the absence of air, to produce carbon materials. The process of pyrolysis results in the removal of volatile organic compounds, such as gases and liquids, from the material, leaving behind a carbon-rich residue.
Pyrolysis is important for many chemical synthesis and battery materials because it is a cost-effective way to produce high-quality carbon materials. The resulting carbon materials, such as carbon black, can be used as raw materials for the production of graphite, graphene, and other advanced carbon materials.
In the field of energy storage, pyrolysis is used to produce carbon materials for use in lithium-ion batteries. The carbon materials produced through pyrolysis have high electrical conductivity and mechanical strength, which make them well-suited for use as electrodes in lithium-ion batteries. In addition, pyrolysis can be used to produce high-purity carbon materials, which are critical for the production of high-performance battery materials.
Pyrolysis is an important process in the production of carbon materials, and is critical for the production of many advanced chemical synthesis and battery materials. The high-quality carbon materials produced through pyrolysis are essential for a wide range of industrial applications, including energy storage and advanced materials.
A furnace is essential for the pyrolysis process because it provides the controlled environment necessary to heat the material to the required temperature in the absence of air. The furnace must be capable of maintaining a temperature of several hundred to several thousand degrees Celsius and must also provide a means of controlling the atmosphere within the furnace to prevent oxidation of the material.
The use of a furnace for pyrolysis provides several advantages over other heating methods, including the ability to control the temperature and atmosphere of the process, the ability to heat the material evenly and consistently, and the ability to carry out the process on a large scale.